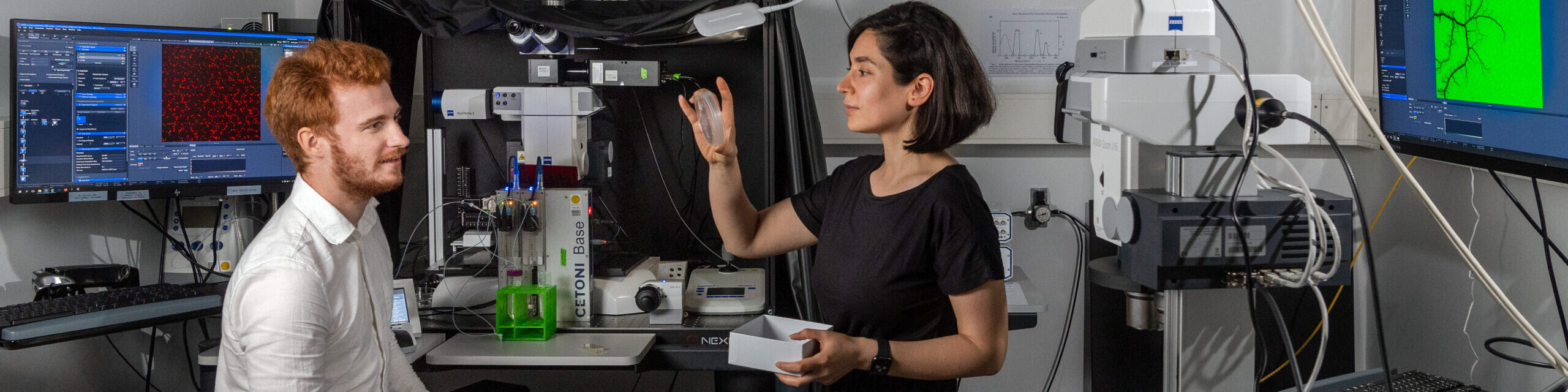
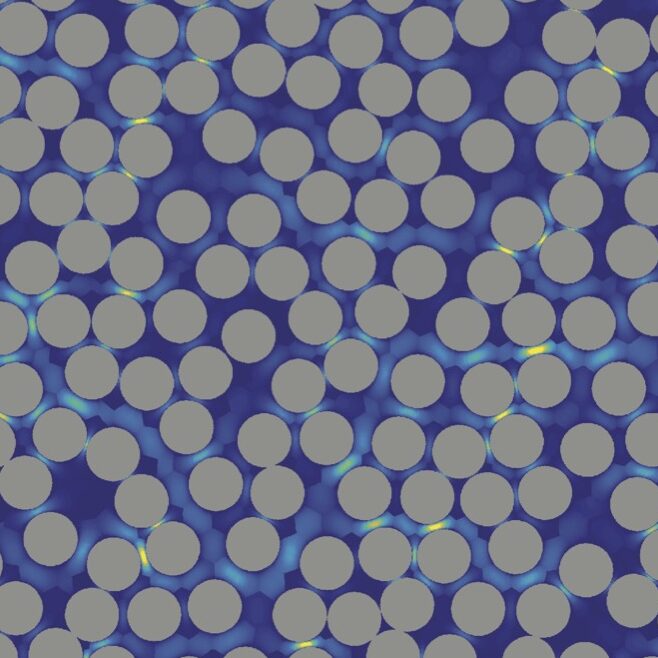
Flow velocity, tube and network architecture, and reaction kinetics act together to control transport along tubes and entire networks.
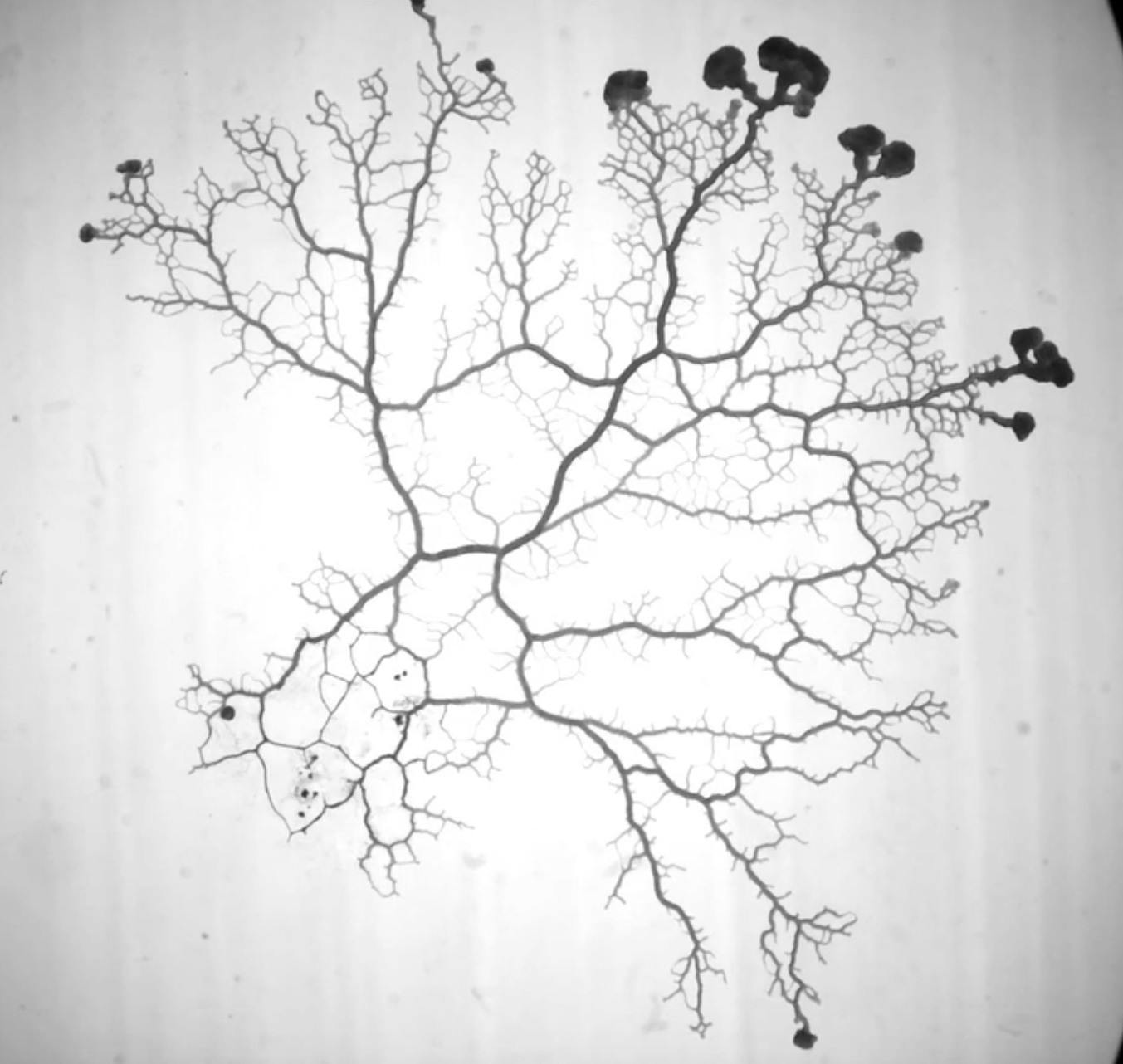
Active flow networks like our vasculature adapt in network architecture and function to the flow and resources pervading them.
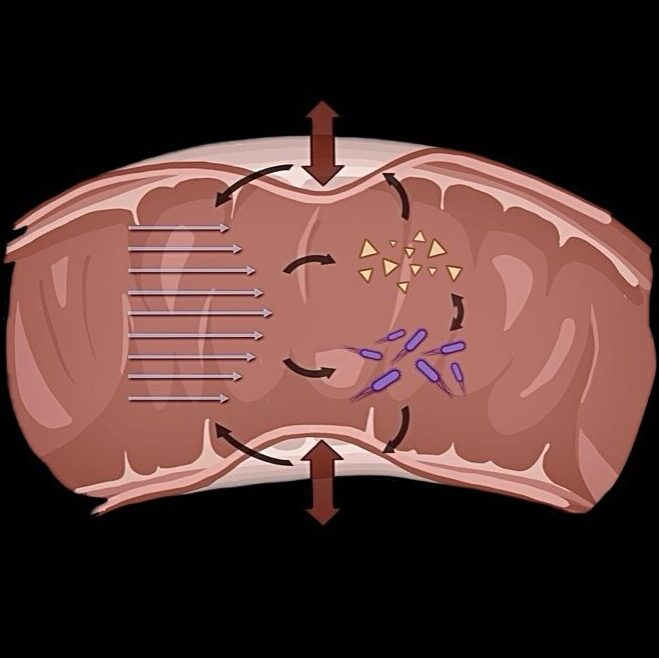
Mechanical properties change in response to (bio-)chemical or mechanical signals thereby self-organizing tissue layout and organ function.